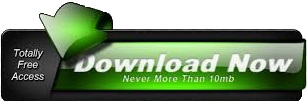

One of the earliest such "plasma guns" was the Marshall Gun, 2 developed at Los Alamos Laboratories in 1960, and which could accelerate a hydrogen plasma to 150 km/s. For even larger currents, some rail guns replace the conducting rod with an ionized plasma, which also conducts electricity. This current may still be too much for a thin wire to take without melting its insulation, but it can be suitable for larger setups. To achieve the same propulsion as we saw with the ceramic magnets, we would need hundreds of amps of current (not tens of thousands of amps). However, as we increase the current, both the magnetic field from the rod and the magnetic field from the rails increases, so that the strength of the push goes up like the square of the current. Unfortunately, for a small battery-powered experiment, the magnetic field is still only a few times stronger than the Earth's magnetic field, too weak to do much good. The rod on the inside sees an upward pointing magnetic field much like in figure 1, and the force on the rod is once again in the forward direction (green arrow).

In this figure, the field lines point down on the outside of the rails, and up on the inside of the rails. According to Ampere's law, which describes the relation between the current and the magnetic field produced, the magnetic field lines form circles around the rails, as shown in figure 2. The rails themselves produce a magnetic field due to the current flowing in them. The Earth is not the only source of magnetic field in this experiment, however. To get the same propulsion using the Earth's field, we would need to put tens of thousands of amps of current through the system, and the metal would probably melt. The issue is that the Earth's magnetic field is tens of thousands of times weaker than the field near a strong ceramic magnet. 1 In Antarctica, the field would look the same as the field in figure 1. Earth's magnetic field is always present, and generally has a component of the field perpendicular to the Earth's surface. In principle, the rail gun can work even without the ceramic magnets. A long enough track will get the rod up to very high speeds, which is probably why it's called a rail gun. The rod will accelerate in that direction. In figure 1, the magnetic field is upwards, the (positive) current flows horizontally through the rod from left to right, and the force on the rod is in the forward direction (green arrow). (Remember that the conventional positive current is defined to be opposite the flow of electrons, and remember that the magnetic field points away from the north pole of a magnet.) Your extended right thumb now points in the direction of the Lorentz force. Place the extended fingers of your right hand in the direction of the current flow and orient your palm in the direction of the magnetic field. To calculate the exact direction of the Lorentz force, we use the right hand rule mnemonic. (See A Survey of Simple Electric Motors for more on the Lorentz force.) For any conducting rod or wire, it's easiest just to remember that the force is always sideways (perpendicular) to the conductor. The force that the current-carrying rod feels in the magnetic field is called a Lorentz force, which is perpendicular to both the magnetic field and the current.

As the current flows through the rod, it produces a magnetic field, which in turn interacts with the magnetic field from the block magnets and gives rise to forces between the rod and the magnets in the same way that two magnets push or pull on each other. Underneath the rod is a set of block magnets with their north poles facing up, producing the magnetic field indicated by the blue arrows. An electric current runs down one rail, through the metal rod and back along the second rail. design 1 - operationįigure 1 shows a schematic of the rail gun arrangement. 2: Schematic of the rail gun with the magnetic field lines from the current in the rails.
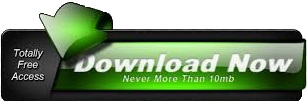